top of page
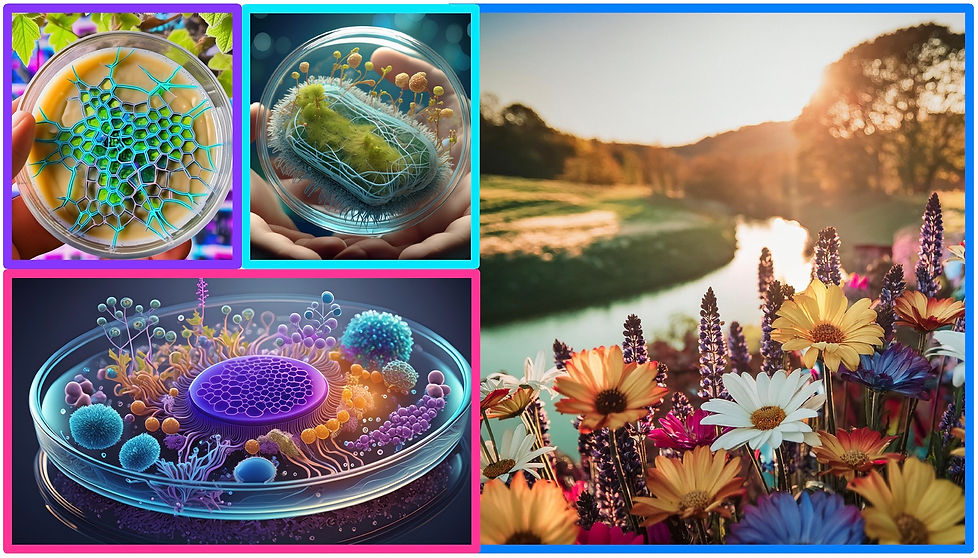
Cegelski Lab
Stanford University
Harnessing chemistry in nature and our imagination for a brighter future.
Bold discovery and new chemistry for urgent problems in need of creative solutions.
We are intrepid explorers and inspired by the challenge and need for solutions to pressing problems in human health, plant and ocean life, and sustainability. We design and deploy uniquely enabling problem-solving approaches integrating chemistry, microbiology, chemical biology, biophysics, and biochemistry to address these grand challenges. We have launched a collaborative antibacterial drug design program integrating synthesis, chemical biology, and mechanistic biochemistry and biophysics directed at the discovery and development of new antibacterial therapeutics targeting difficult-to-treat bacteria.
We recently identified a new chemical structure never before observed in nature: phosphoethanolamine (pEtN) cellulose. We also uncovered the genetic and molecular basis for its installation, identifying a new pEtN transferase, BcsG. Common E. coli and other bacteria cloak themselves in pEtN cellulose - "a bacterial coat that is not pure cotton." This modified cellulose in commonly studied microbes evaded detection for decades. We are discovering such dark matter that poses a challenge to discovery and analysis by conventional methods.
We interrogate and map the molecular, spatial and temporal dynamics in bacterial biofilms that include pEtN cellulose as well as fascinating extracellular functional amyloid fibers termed curli and other matrix components. We strive to understand at a molecular and atomic level how cells self-assemble fascinating cell walls, extracellular structures, and how bacteria use these building blocks to construct organized biofilm architectures. We are also engaged in identifying small molecules to interfere with these processes, inhibiting cell-wall biosynthesis, amyloid assembly and polysaccharide assembly to introduce new antibacterial and antibiotic-sparing anti-virulence therapeutics for infectious diseases and promising compounds to interfere with neurodegenerative disease.
Cegelski Lab
Department of Chemistry | Stanford University
bottom of page